No products in the cart.
Longevity - Oxidative Stress
Redox balance refers to the state of equilibrium between oxidation and reduction reactions within a cell or organism. These chemical reactions are crucial for energy production, detoxification, and cellular signaling processes. Oxidative stress occurs when there's an imbalance in this system
- Test Requisition Form
- Sample Report
- Blood Collection Video
- Brochure
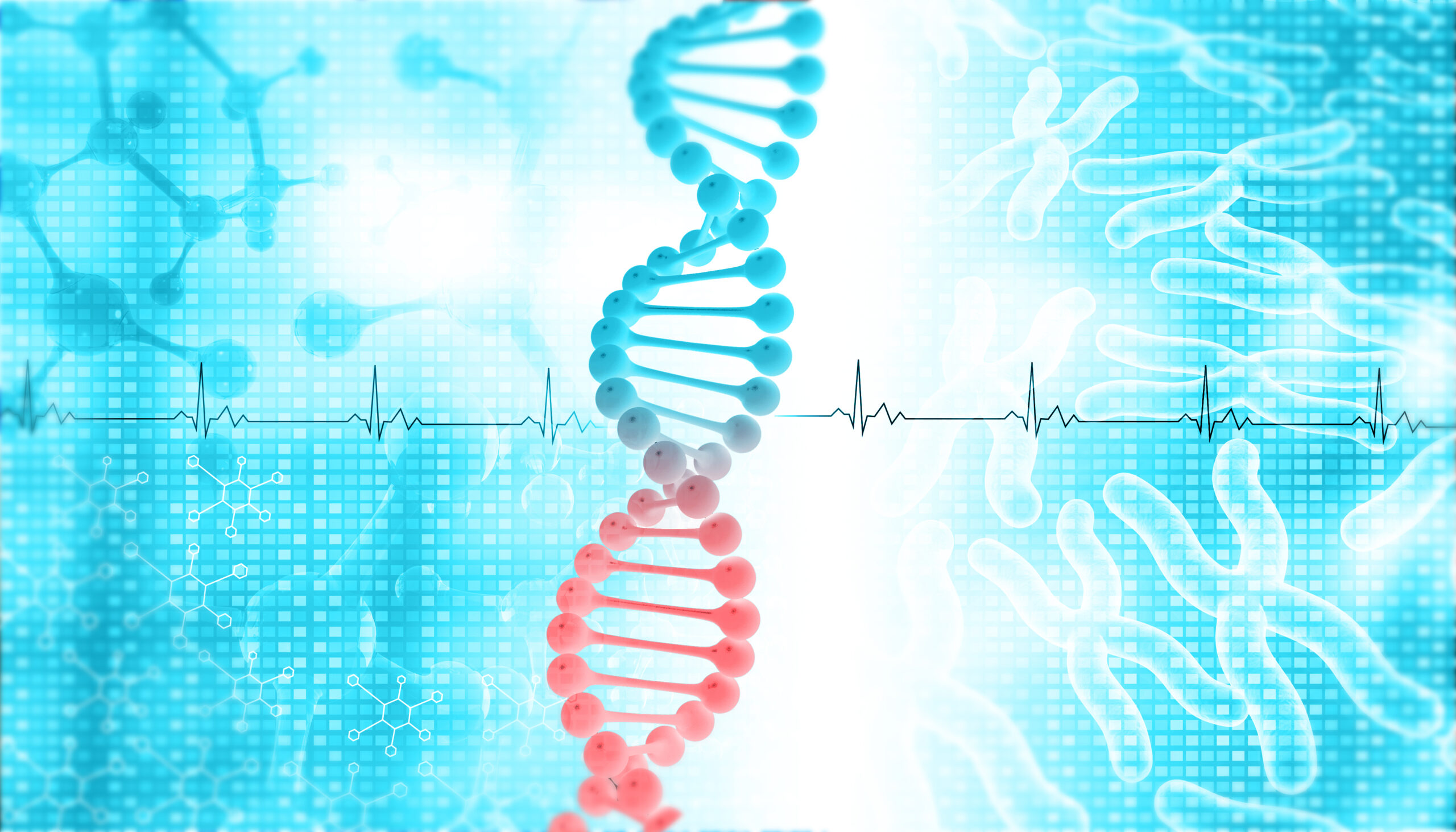
REDOX Balance And Oxidative Stress
Redox balance and oxidative stress are central themes in the study of longevity and the aging process. Redox balance refers to the state of equilibrium between oxidation and reduction reactions within a cell or organism. These chemical reactions are crucial for energy production, detoxification, and cellular signaling processes. Oxidative stress occurs when there’s an imbalance in this system—specifically, when the production of reactive oxygen species (ROS) exceeds the body’s ability to neutralize and eliminate them.
Redox Balance: At the core of cellular metabolism lies the redox balance, a critical equilibrium between oxidation (loss of electrons) and reduction (gain of electrons) reactions. This balance is vital for maintaining cellular function and integrity. Redox reactions are involved in energy production, detoxification, cellular signaling, and the immune response. The body employs an array of antioxidant mechanisms to maintain redox homeostasis, neutralizing excess reactive oxygen species (ROS) and preventing cellular damage.
Oxidative Stress: Oxidative stress arises when there’s a disruption in redox homeostasis, with the scale tipping towards excess ROS production. While ROS are natural by-products of oxygen metabolism and play roles in cell signaling and homeostasis, excessive ROS levels can damage proteins, lipids, and DNA, contributing to cellular aging and the onset of age-related diseases such as cardiovascular diseases, diabetes, neurodegenerative disorders, and cancer.
Mitochondrial dysfunction is closely related to this imbalance as mitochondria are the primary site of energy production and significant generators of ROS. When mitochondria function improperly, it can lead to increased oxidative stress, which in turn can damage cellular components and contribute to various diseases and aging processes. Maintaining redox balance is therefore vital for cellular health and overall well-being.
7 Analytes Tested: Glutathione, Malondialdehyde (MDA), Reactive Oxygen Species (ROS), Superoxide Dismutases (SODs), Advanced Glycation End Products (AGES), Total Antioxidant Capacity (TAC), 8-Hydroxy-2′-deoxyguanosine (8-OHdG)
Price: $299.00
Price includes a convenient home collection kit for sample collection from the comfort of your home
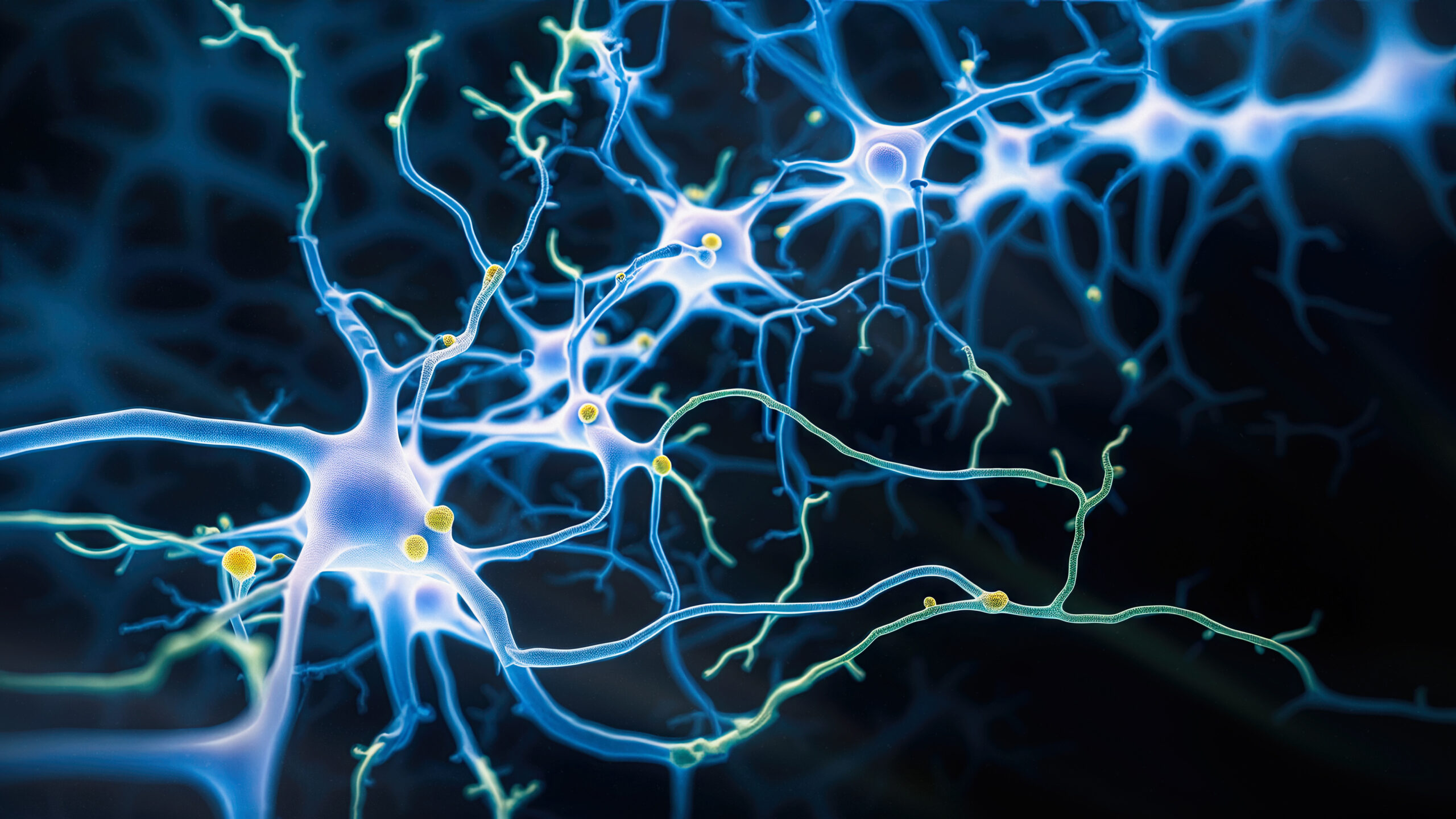
Reactive Oxygen Species (ROS)
Reactive oxygen species (ROS) are chemically reactive molecules containing oxygen. They are natural byproducts of normal cellular metabolism and are involved in various physiological processes. However, excessive production of ROS can lead to oxidative stress, which can damage cells and tissues. Here’s an overview of reactive oxygen species:
Types of Reactive Oxygen Species
- Superoxide Radicals (O2•-): Formed during normal cellular metabolism, particularly in the mitochondria.
- Hydrogen Peroxide (H2O2): A non-radical ROS that can diffuse across membranes and can be converted to highly reactive hydroxyl radicals.
- Hydroxyl Radicals (•OH): Among the most reactive ROS, formed through the Fenton reaction involving hydrogen peroxide and transition metals like iron (Fe) or copper (Cu).
Sources of Reactive Oxygen Species
- Mitochondrial Respiration: The electron transport chain in mitochondria produces ROS as a byproduct during ATP (energy) production.
- Inflammation: Immune cells produce ROS to kill pathogens during immune responses.
- Environmental Factors: Exposure to pollutants, radiation (UV rays), and certain chemicals can increase ROS production.
Role in Disease and Aging
- Oxidative Stress: Excessive ROS production can overwhelm antioxidant defenses, leading to oxidative damage to lipids, proteins, and DNA, implicated in various diseases such as cardiovascular disease, neurodegenerative diseases (like Alzheimer’s and Parkinson’s), cancer, and aging processes.
Regulation and Control
- Antioxidants: Consuming antioxidants (from fruits, vegetables, and supplements) can help neutralize ROS and reduce oxidative stress.
- Enzymatic Systems: Cells use antioxidant enzymes to scavenge ROS and maintain cellular homeostasis.
Conclusion
While reactive oxygen species play essential roles in normal physiological functions, their overproduction and accumulation can lead to oxidative stress and contribute to various diseases. Maintaining a balance between ROS production and antioxidant defenses is crucial for cellular health and overall well-being.
Price: $99.00
Oxidative Stress And Longevity
Oxidative stress plays a complex role in the aging process and longevity. It occurs when there’s an imbalance between the production of reactive oxygen species (ROS) and the body’s ability to detoxify these reactive intermediates or to repair the resulting damage. ROS are by-products of normal cellular metabolic processes, but excessive ROS can lead to cell and tissue damage, contributing to aging and the development of age-related diseases such as cardiovascular diseases, diabetes, cancer, and neurodegenerative disorders.
However, the relationship between oxidative stress and longevity is not straightforward. While high levels of oxidative stress can accelerate the aging process and contribute to disease, a moderate increase in ROS can actually have beneficial effects through a process known as hormesis. Hormesis refers to a biological phenomenon where a beneficial effect (such as improved health, stress tolerance, growth, or longevity) results from exposure to low doses of an agent that is otherwise toxic or lethal when given at higher doses. In the context of oxidative stress, low levels of ROS can stimulate protective, adaptive responses in cells that enhance their ability to cope with greater stresses, potentially leading to increased lifespan and healthspan.
To reiterate this:- Paradoxically, while excessive oxidative stress is detrimental, a certain level of ROS is necessary for the activation of adaptive stress response pathways that enhance cellular defense mechanisms and longevity. This concept, known as hormesis, suggests that low-level exposure to stressors that produce ROS can promote longevity through the activation of signaling pathways that improve the organism’s stress resistance and repair mechanisms.
Additionally, the body’s antioxidative defense mechanisms, which include enzymes like superoxide dismutase (SOD), catalase, and glutathione peroxidase, play a critical role in maintaining redox balance and protecting against oxidative damage. The effectiveness of these defense mechanisms is a key determinant of lifespan and a focal point of research on aging and longevity.
Mitochondrial Dysfunction And Longevity
Mitochondria, the energy-producing structures within cells, are essential for life and health. Mitochondrial dysfunction can cause numerous health problems and has been linked to a variety of diseases, including neurodegenerative diseases, cardiovascular disease, diabetes, and cancer. Mitochondrial dysfunction has also been associated with the aging process and may affect longevity. Here’s how:
Mitochondrial DNA Damage: Mitochondria have their own DNA (mtDNA), separate from the nuclear DNA found in the cell nucleus. Because mitochondria are the site of oxidative metabolism, mtDNA is more exposed to oxidative damage. Over time, the accumulation of these damages can impair mitochondrial function, leading to decreased cellular function and increased cell death.
Reactive Oxygen Species (ROS) Production: Mitochondria produce reactive oxygen species (ROS) as a byproduct of energy (ATP) production. While ROS play important roles in cell signaling and homeostasis, excessive ROS can damage cell structures and DNA. This oxidative stress can lead to cellular dysfunction, aging, and various diseases.
Mitophagy and Cellular Quality Control: Mitochondria undergo a process known as mitophagy, a type of autophagy where damaged or dysfunctional mitochondria are degraded and recycled. With age, the efficiency of mitophagy can decline, leading to an accumulation of damaged mitochondria, which can further increase ROS production and cellular damage.
Bioenergetic Decline: With age, the efficiency of the mitochondrial electron transport chain, which generates the majority of cellular ATP, can decrease. This decline in mitochondrial bioenergetics can lead to reduced cellular energy levels, which can contribute to the development of age-related diseases and impact longevity.
Role in Apoptosis: Mitochondria play a key role in apoptosis (programmed cell death). Mitochondrial dysfunction can disrupt this process, leading to either excessive cell death or the survival of cells with significant damage, both of which can contribute to aging and disease.
In conclusion, maintaining mitochondrial health is critical for cellular function, and mitochondrial dysfunction is a key component of the aging process. It’s also important to note that while aging is associated with an overall decline in mitochondrial function, there are vast differences between individuals and across different tissues within the same individual.
Researchers are investigating several ways to combat mitochondrial dysfunction, such as promoting mitochondrial biogenesis, enhancing the efficiency of the electron transport chain, reducing oxidative damage, and improving mitophagy. However, the development of interventions that can effectively target mitochondria to improve health and extend lifespan is still a significant challenge in the field of aging research.
Test Details
Redox Balance and Oxidative Stress are central themes in the study of longevity and the aging process. The intricate balance between these two states within the body significantly impacts cellular health, aging, and lifespan. Measuring redox balance and oxidative stress through blood tests involves quantifying substances that either contribute to or protect against the imbalance of oxidants and antioxidants in the body.
14 Analytes Tested
- GSH/GSSG
- 8-Isoprostane
- Malondialdehyde (MDA)
- NAD/NADH
- inducible Nitric Oxide Synthase (iNOS)
- Reactive Oxygen Species (ROS)
- Peroxidases
- Superoxide Dismutases (SODs)
- Advanced Oxidation Protein Products (AOPP)
- Total Antioxidant Capacity (TAC)
- Protein Carbonyls
- 8-Hydroxy-2′-deoxyguanosine (8-OHdG)
Glutathione (GSH/GSSG) Ratio: The ratio of reduced glutathione (GSH) to oxidized glutathione (GSSG) in blood is a direct measure of cellular oxidative stress and redox balance. A low GSH/GSSG ratio indicates oxidative stress.
The Glutathione (GSH/GSSG) ratio is a critical indicator of cellular health, oxidative stress, and the overall redox state within an organism. Glutathione, a tripeptide consisting of the amino acids glutamine, cysteine, and glycine, exists in two forms within cells: the reduced form (GSH) and the oxidized form (GSSG). The balance between these two forms is essential for maintaining cellular integrity and function.
- Role of GSH and GSSG:
- GSH (Reduced Glutathione): Acts as a major antioxidant by directly scavenging reactive oxygen species (ROS) and by serving as a substrate for glutathione peroxidase, an enzyme that reduces peroxides. GSH also plays a role in detoxification processes, the regeneration of other antioxidants, and maintaining the redox status of protein thiols.
- GSSG (Oxidized Glutathione): Formed when two GSH molecules oxidize and bond together through a disulfide bridge. The body can recycle GSSG back to GSH through the action of glutathione reductase, using NADPH as a reducing agent, thus sustaining the antioxidant capacity of cells.
- GSH/GSSG Ratio and Oxidative Stress:
- The GSH/GSSG ratio is often used as a measure of cellular oxidative stress and the redox environment. A high ratio indicates a reductive (antioxidant-rich) environment, whereas a low ratio signifies oxidative stress, where the production of ROS overwhelms the antioxidant defense mechanisms, leading to potential cellular damage.
- Oxidative stress, marked by a decreased GSH/GSSG ratio, is implicated in the pathogenesis of numerous diseases, including cancer, cardiovascular diseases, neurodegenerative disorders, and aging processes. It results in damage to critical cellular components such as lipids, proteins, and DNA.
- Implications and Interventions:
- Understanding the GSH/GSSG ratio’s role in health and disease has led to the exploration of therapeutic interventions aimed at enhancing glutathione levels or improving the GSH/GSSG ratio. These include dietary supplements (e.g., N-acetylcysteine, whey protein), lifestyle modifications, and pharmacological agents that either boost GSH synthesis, reduce GSSG, or enhance the efficiency of the glutathione recycling system.
- In summary, the GSH/GSSG ratio is a fundamental marker of cellular oxidative stress and an important determinant of the body’s ability to counteract ROS-induced damage. Maintaining an optimal GSH/GSSG ratio is crucial for cellular health, disease prevention, and healthy aging.
8-Isoprostane, specifically 8-iso-Prostaglandin F2α (8-iso-PGF2α), is a biomarker of oxidative stress that is considered one of the most reliable indicators of lipid peroxidation in vivo. Isoprostanes are prostaglandin-like compounds formed independently of the cyclooxygenase enzymes, through the free radical-catalyzed peroxidation of arachidonic acid embedded within cell membranes.
Key Aspects of 8-Isoprostane and Oxidative Stress:
- Formation: 8-Isoprostanes are produced when ROS attack polyunsaturated fatty acids, specifically arachidonic acid, leading to lipid peroxidation. The process is non-enzymatic and can occur under conditions of oxidative stress.
- Indicator of Oxidative Damage: The level of 8-isoprostane is a direct measure of oxidative damage to lipids, reflecting the extent of lipid peroxidation. Elevated levels indicate higher oxidative stress, which is implicated in the pathophysiology of various diseases.
- Association with Diseases: Increased 8-isoprostane levels have been linked to a wide range of conditions, including cardiovascular diseases, pulmonary diseases (such as asthma and chronic obstructive pulmonary disease), metabolic disorders (like diabetes), neurological diseases, and aging. It serves as an important marker for assessing disease risk, progression, and the effectiveness of antioxidant therapies.
- Advantages Over Other Biomarkers: One of the advantages of 8-isoprostane as a biomarker is its greater stability and specificity for lipid peroxidation.
Malondialdehyde (MDA): MDA is a byproduct of lipid peroxidation and serves as a marker for oxidative stress. Elevated levels of MDA in the blood are indicative of increased lipid peroxidation.
Malondialdehyde (MDA) is a well-known biomarker of oxidative stress and lipid peroxidation. Lipid peroxidation is a process in which free radicals, particularly reactive oxygen species (ROS), attack lipids containing carbon-carbon double bonds in cell membranes, leading to cell damage. MDA is one of the final products of the polyunsaturated fatty acids peroxidation in the cells and is often used to estimate the level of oxidative stress in an organism.
Relationship between MDA and Oxidative Stress:
- Indicator of Lipid Peroxidation: MDA is considered a reliable indicator of lipid peroxidation. The accumulation of MDA in tissues or biological fluids signifies oxidative damage to cell membranes, which can affect cell viability and function.
- Association with Diseases: Elevated levels of MDA are associated with various diseases and conditions characterized by oxidative stress, including cardiovascular diseases, diabetes, cancer, neurodegenerative disorders, and aging. Monitoring MDA levels can help in assessing the severity of disease processes and the effectiveness of antioxidant therapy.
- Role in Research and Clinical Practice: Research into MDA and oxidative stress contributes to our understanding of the pathophysiology of oxidative damage and its implications for health and disease. In clinical practice, measuring MDA levels can aid in diagnosing and monitoring conditions associated with oxidative stress.
The Nicotinamide Adenine Dinucleotide (NAD⁺/NADH) ratio is a crucial indicator of cellular redox balance, playing a significant role in metabolism and the regulation of oxidative stress. NAD⁺ and its reduced form, NADH, are coenzymes involved in numerous biochemical reactions, including those in the mitochondrial electron transport chain where cellular energy (ATP) is produced.
- NAD⁺/NADH in Redox Balance:
- Redox Reactions: NAD⁺ acts as an electron acceptor in redox reactions, converting to NADH when it gains electrons (reduced). Conversely, NADH can donate these electrons (oxidized) to other molecules, turning back into NAD⁺. This cycle is integral to energy metabolism, particularly in glycolysis, the Krebs cycle, and oxidative phosphorylation.
- Energy Production: In mitochondria, NADH donates electrons to the electron transport chain, a process essential for the production of ATP. The efficiency of this process and the balance between NAD⁺ and NADH influence cellular energy levels and overall metabolic health.
- Regulation of Oxidative Stress: NAD⁺ plays a role in modulating oxidative stress. It serves as a substrate for sirtuins (SIRTs) and poly(ADP-ribose) polymerases (PARPs), enzymes involved in DNA repair, stress responses, and inflammation. High NAD⁺ levels enhance the activity of these enzymes, promoting cell survival under stress conditions.
- NAD⁺/NADH Ratio and Oxidative Stress:
- A balanced NAD⁺/NADH ratio is vital for maintaining cellular health. A high ratio generally indicates good mitochondrial function and efficient energy production, while a low ratio can signify metabolic disturbances and increased oxidative stress.
- Conditions of oxidative stress are often associated with a reduced NAD⁺/NADH ratio, reflecting either an excessive consumption of NAD⁺ or inadequate regeneration of NAD⁺ from NADH. This imbalance can impair cellular functions, including energy production and the ability to respond to oxidative damage.
- Oxidative stress and altered NAD⁺/NADH ratios are linked to aging and various diseases, such as neurodegenerative disorders, cardiovascular diseases, and metabolic conditions like diabetes.
- In summary, the NAD⁺/NADH ratio is a key determinant of cellular redox state, energy metabolism, and the body’s capacity to handle oxidative stress. Maintaining an optimal ratio is essential for metabolic health, and interventions that can boost NAD⁺ levels or improve the efficiency of NAD⁺/NADH cycling hold promise for mitigating oxidative stress and enhancing cellular resilience.
iNOS is an enzyme responsible for the production of nitric oxide (NO) in response to inflammatory stimuli. While NO has various physiological roles, its overproduction can lead to oxidative stress:
- NO and ROS: Although NO itself is a free radical, its interactions with superoxide lead to the formation of peroxynitrite, a potent oxidant capable of inducing significant cellular damage, including lipid peroxidation, DNA damage, and protein modification.
- Inflammatory Conditions: iNOS-induced NO production is elevated in various inflammatory and autoimmune conditions, contributing to the pathophysiology of diseases by exacerbating oxidative stress and tissue damage.
- Regulation of iNOS: Given its potential for contributing to oxidative stress, the regulation of iNOS expression and activity is a target for therapeutic interventions in diseases characterized by chronic inflammation and oxidative damage. Antioxidants and specific inhibitors of iNOS are explored for their potential to mitigate these effects.
Advanced Oxidation Protein Products (AOPP): AOPPs are markers of oxidative damage to proteins and can indicate oxidative stress levels and antioxidant defense capacity.
Advanced Oxidation Protein Products (AOPP) are dityrosine-containing cross-linked protein products formed during oxidative stress through the reaction of plasma proteins with chlorinated oxidants, such as hypochlorous acid (HOCl) and chloramines, produced by myeloperoxidase in activated neutrophils. AOPPs are considered reliable markers for assessing the degree of protein oxidation and are closely associated with chronic inflammation and oxidative stress.
- Relationship Between AOPP and Oxidative Stress:
- Indicator of Protein Oxidation: AOPPs directly reflect oxidative damage to proteins, a key aspect of oxidative stress. They accumulate in various pathological conditions associated with increased oxidative stress and inflammation.
- Association with Diseases: Elevated levels of AOPPs have been linked to a wide range of diseases, including chronic kidney disease, cardiovascular diseases, diabetes, rheumatoid arthritis, and neurodegenerative disorders. High AOPP levels indicate an imbalance between oxidative forces and the antioxidative defense mechanism, leading to tissue damage and progression of disease.
- Aging and Oxidative Stress: Aging is associated with increased oxidative stress, and AOPPs have been used as biomarkers to study the oxidative status in aging populations. Their accumulation is a sign of the oxidative damage that accumulates over time, contributing to the aging process and age-related diseases.
- In summary, AOPPs serve as important biomarkers for oxidative stress and protein oxidation, offering insights into the extent of oxidative damage in the body. Understanding and managing oxidative stress through various interventions can help mitigate the progression of related diseases and promote overall health and longevity.
Peroxidases are a group of enzymes that play a crucial role in managing oxidative stress by catalyzing the breakdown of peroxides, particularly hydrogen peroxide (H2O2), into water and oxygen. This action helps to mitigate the potential harmful effects of reactive oxygen species (ROS) on cells, including damage to proteins, lipids, and DNA. The presence and activity of peroxidases in cells are vital for maintaining cellular health and protecting against oxidative damage.
- Key Functions of Peroxidases:
- Detoxification of Peroxides: Peroxidases reduce H2O2, a common ROS that can be produced during normal metabolic processes or due to environmental stressors. By converting H2O2 into water and oxygen, peroxidases prevent the accumulation of H2O2 and its conversion into more harmful radicals, such as hydroxyl radicals.
- Antioxidant Defense: These enzymes are an integral part of the cell’s antioxidant defense system. Alongside other antioxidant enzymes like superoxide dismutase (SOD) and catalase, peroxidases contribute to the detoxification of ROS and protection against oxidative stress.
- Signaling: Beyond detoxification, H2O2 serves as a signaling molecule involved in various cellular processes, including growth, apoptosis, and the immune response. Peroxidases help regulate the concentration of H2O2, ensuring that signaling processes are properly modulated without causing oxidative damage.
- Types of Peroxidases and Their Impact on Oxidative Stress:
- Glutathione Peroxidase (GPx): Uses glutathione (GSH) as a substrate to reduce H2O2, forming water and oxidized glutathione (GSSG). GPx is crucial for protecting cellular components from oxidative damage.
- Catalase: Catalyzes the decomposition of H2O2 to water and oxygen at a very high rate, particularly effective at higher concentrations of H2O2. Found in peroxisomes of virtually all aerobic cells, catalase is essential for controlling oxidative stress in cells.
- Myeloperoxidase (MPO): While MPO plays a role in the immune response by producing hypochlorous acid (HOCl) from H2O2, it can also contribute to tissue damage and inflammation if excessively activated, indicating a dual role in both defense and promotion of oxidative stress.
- Peroxidases and Oxidative Stress:
- Protective Role: By reducing H2O2, peroxidases help to prevent the formation of more reactive oxygen species, such as hydroxyl radicals, which can cause significant cellular damage. This action is vital for maintaining cellular integrity and preventing oxidative stress-related diseases.
- Indicator of Oxidative Stress: Elevated levels of peroxidase enzymes, especially in the blood, can serve as indicators of oxidative stress and inflammation within the body. For example, increased MPO levels have been associated with cardiovascular diseases.
- Therapeutic Targets: Given their role in managing oxidative stress, peroxidases and their pathways are considered potential therapeutic targets. Enhancing the activity of GPx and catalase or modulating MPO activity can provide strategies to mitigate oxidative stress and its associated pathologies.
- In summary, peroxidases play a critical role in cellular defense against oxidative stress by catalyzing the reduction of peroxides. Their activity is essential for preventing damage to cellular components and maintaining health. Understanding the balance and regulation of peroxidase activities provides insights into the mechanisms of oxidative stress and opportunities for therapeutic intervention in diseases characterized by excessive oxidative damage.
Protein carbonyls are formed by the oxidation of proteins and serve as markers of oxidative protein damage.
Protein carbonyls are markers of oxidative protein damage and are considered one of the primary indicators of oxidative stress within cells. They form when reactive oxygen species (ROS) or other reactive compounds, such as aldehydes, react with protein side chains, including lysine, arginine, proline, and threonine. This modification not only marks the proteins for degradation but also impairs their function, which can contribute to cellular dysfunction and the pathogenesis of various diseases.
- Formation of Protein Carbonyls:
- Direct Oxidation: Proteins can be directly oxidized by ROS, leading to the formation of carbonyl groups on the amino acid side chains.
Secondary Products: Reactive aldehydes, which can be by-products of lipid peroxidation or glycation processes, can also modify proteins, contributing to the formation of carbonyl derivatives.
- Direct Oxidation: Proteins can be directly oxidized by ROS, leading to the formation of carbonyl groups on the amino acid side chains.
- Implications of Protein Carbonylation:
- Cellular Dysfunction: Carbonylated proteins often lose their functional activity, contributing to impaired cellular processes, enzyme inactivation, and loss of structural integrity.
- Aging and Disease: Accumulation of carbonylated proteins is associated with aging and numerous age-related diseases, including neurodegenerative disorders (like Alzheimer’s and Parkinson’s disease), cardiovascular diseases, diabetes, and cancer. The presence of protein carbonyls is a sign of oxidative damage and cellular stress.
- Cellular Response: Cells attempt to cope with protein carbonylation through various mechanisms, including the degradation of damaged proteins by proteasomes and autophagy. However, persistent or excessive carbonylation can overwhelm these systems, leading to cell death or senescence.
Reactive oxygen species (ROS) are highly reactive molecules that are generated as byproducts of normal cellular metabolism, as well as during inflammation and immune responses. While ROS play important roles in cell signaling and host defense, their excessive or uncontrolled production can contribute to inflammation. Here’s how ROS and inflammation are interconnected:
ROS as Signaling Molecules: In low to moderate concentrations, ROS can act as signaling molecules involved in various cellular processes, including inflammation. ROS can regulate the activation and migration of immune cells, gene expression, and cytokine production. They can activate redox-sensitive transcription factors, such as nuclear factor-kappa B (NF-κB) and activator protein-1 (AP-1), which play key roles in initiating inflammatory responses.
Immune Cell Activation and ROS Production: During immune responses, immune cells such as neutrophils, macrophages, and dendritic cells produce ROS as part of their antimicrobial defense mechanisms. ROS production is tightly regulated and serves to kill invading pathogens. However, excessive or dysregulated ROS production can result in collateral damage to surrounding tissues and promote inflammation.
Oxidative Stress and Inflammation: When the balance between ROS production and the body’s antioxidant defense mechanisms is disrupted, a condition known as oxidative stress occurs. Oxidative stress can arise from factors such as environmental toxins, chronic infections, and inflammation itself. Excessive ROS production and inadequate antioxidant capacity can lead to damage to proteins, lipids, and DNA, which triggers inflammatory responses and further exacerbates inflammation.
Inflammatory Diseases and ROS: Chronic inflammatory conditions, such as rheumatoid arthritis, inflammatory bowel disease, and atherosclerosis, are characterized by sustained inflammation and increased production of ROS. In these diseases, ROS contribute to tissue damage, perpetuate inflammatory responses, and promote the activation and migration of immune cells. ROS-induced oxidative stress can also lead to activation of inflammatory signaling pathways, contributing to chronic inflammation.
Antioxidants and Inflammation: Antioxidants, both endogenous (produced by the body) and exogenous (obtained from diet or supplements), play a crucial role in modulating ROS and inflammation. Antioxidants neutralize ROS and help maintain redox balance, thereby mitigating excessive inflammation and reducing tissue damage. Dietary sources of antioxidants, such as fruits, vegetables, and certain spices, have been associated with anti-inflammatory effects.
It’s important to note that ROS and inflammation have complex interactions, and the roles of ROS in inflammation can vary depending on the context and specific conditions. While excessive ROS production can contribute to chronic inflammation, controlled ROS generation is essential for normal immune responses and tissue repair. Balance is crucial, and excessive ROS or antioxidant supplementation should be approached with caution.
If you have concerns about ROS, inflammation, or related conditions, it’s advisable to consult with a healthcare professional who can evaluate your specific situation, perform appropriate tests, and provide personalized advice and guidance.
Superoxide Dismutases (SODs) are a crucial family of enzymes that play a vital role in the body’s defense against oxidative stress. They catalyze the dismutation of the superoxide radical (O2•-) into oxygen (O2) and hydrogen peroxide (H2O2), effectively converting a harmful reactive oxygen species (ROS) into less dangerous substances. Given that the superoxide radical is one of the primary ROS generated in cells, especially during mitochondrial electron transport, SODs are essential for protecting cellular components from oxidative damage.
- Types of SODs: There are three main types of SODs, distinguished by their metal cofactors and locations within the cell:
- Cu/Zn SOD (SOD1): Primarily located in the cytoplasm, this enzyme contains copper and zinc ions and protects cell structures from oxidative damage by superoxide radicals generated within the cytoplasm.
- Mn SOD (SOD2): Found in the mitochondria, this enzyme has manganese ions and is particularly important for neutralizing superoxide radicals produced as a byproduct of mitochondrial respiration, thus preventing mitochondrial DNA damage and dysfunction.
- EC-SOD (SOD3): This form, containing copper and zinc ions like SOD1, is extracellular and protects tissues from oxidative damage by removing superoxide radicals in the extracellular space.
- SODs and Oxidative Stress:
- Protective Role: By converting superoxide radicals into hydrogen peroxide, SODs reduce the potential for these radicals to participate in harmful reactions, such as the Fenton reaction, which produces the highly reactive hydroxyl radical. Although H2O2 is also reactive, it is less damaging than superoxide and is further reduced to water by other antioxidant enzymes, such as catalase and glutathione peroxidase.
- Regulation of Cell Signaling: Beyond detoxification, the action of SODs influences cellular signaling pathways that are regulated by ROS. For example, controlled levels of ROS can act as signaling molecules in processes like cell proliferation, differentiation, and response to environmental stimuli. SODs help maintain ROS at levels that support healthy cellular signaling rather than causing damage.
- Implications for Diseases: Dysregulation of SOD activity or expression has been implicated in various diseases characterized by oxidative stress, including neurodegenerative diseases (such as ALS and Parkinson’s disease), cardiovascular diseases, and cancer. Either excessive ROS production due to low SOD activity or mutations in SOD genes can lead to increased oxidative damage and cellular dysfunction.
Total Antioxidant Capacity (TAC): This test measures the overall ability of blood plasma to counteract ROS and protect the body from oxidative damage. It encompasses the cumulative action of all antioxidants present in plasma and body fluids.
- Total Antioxidant Capacity (TAC) is a comprehensive measure reflecting the cumulative effect of all antioxidants present in plasma and body fluids, offering insight into the overall status of the body’s antioxidant defense system against oxidative stress. Oxidative stress arises when there’s an imbalance between the production of reactive oxygen species (ROS) and the body’s ability to neutralize them with antioxidants. This imbalance can lead to cellular damage, contributing to various chronic diseases and aging.
- Importance of TAC in Understanding Oxidative Stress:
- Holistic Indicator: TAC provides a holistic view of the antioxidant defense status rather than focusing on individual antioxidants. It accounts for the synergistic effects among different antioxidants, including enzymes, vitamins, minerals, and phytochemicals.
- Assessment of Disease Risk: Elevated levels of oxidative stress have been linked to numerous conditions such as cardiovascular diseases, diabetes, neurodegenerative diseases, and cancer. TAC measurements can help assess the risk and progression of these diseases by indicating whether antioxidant defenses are sufficient to counteract oxidative stress.
- Evaluation of Dietary and Lifestyle Impact: TAC levels can be influenced by diet, lifestyle, and environmental factors. Diets rich in fruits, vegetables, and whole grains tend to increase TAC, offering protection against oxidative stress. Smoking, alcohol consumption, and exposure to pollutants, on the other hand, can decrease TAC.
- In summary, TAC is a valuable indicator of the body’s overall antioxidant defense capability against oxidative stress. Understanding TAC levels can help in assessing disease risk, evaluating the impact of diet and lifestyle, and guiding interventions to enhance antioxidant defenses and maintain redox balance. However, interpreting TAC requires consideration of the broader context of individual health, lifestyle, and environmental factors.
8-Hydroxy-2′-deoxyguanosine (8-OHdG): A marker of oxidative DNA damage, elevated levels of 8-OHdG in urine or blood are indicative of oxidative stress affecting DNA integrity.
8-Hydroxy-2′-deoxyguanosine (8-OHdG) is a biomarker widely recognized for its significance in measuring oxidative damage to DNA. It results from the modification of guanine bases in DNA by reactive oxygen species (ROS) and serves as a sensitive indicator of oxidative stress at the cellular level. The accumulation of 8-OHdG within cellular DNA is considered a critical event in the pathogenesis of various diseases and aging due to its potential to cause mutations and alter gene expression.
- Role and Implications of 8-OHdG in Oxidative Stress:
- Marker of Oxidative DNA Damage: The presence of 8-OHdG in DNA is a direct consequence of oxidative damage inflicted by ROS. Elevated levels of 8-OHdG are often associated with increased oxidative stress, reflecting the extent of cellular exposure to harmful oxidative conditions.
- Association with Diseases and Aging: Chronic oxidative stress, as indicated by high levels of 8-OHdG, has been linked to the development and progression of numerous diseases, including cancer, cardiovascular diseases, neurodegenerative disorders, and diabetes. It is also associated with the aging process, contributing to the accumulation of genetic mutations and cellular dysfunction over time.
- Environmental and Lifestyle Factors: External factors such as exposure to UV light, radiation, smoking, pollution, and dietary habits can influence the levels of oxidative DNA damage, as measured by 8-OHdG. This highlights the role of environmental and lifestyle choices in modulating oxidative stress and its impact on health.
- Significance and Therapeutic Implications:
- Risk Assessment and Monitoring: Monitoring 8-OHdG levels can help assess the risk of developing oxidative stress-related diseases and evaluate the effectiveness of interventions aimed at reducing oxidative damage, such as antioxidant supplementation or lifestyle modifications.
- Antioxidant Defense: Understanding the mechanisms leading to oxidative DNA damage and the body’s response to it can inform strategies to enhance antioxidant defenses, potentially mitigating the adverse effects of ROS on DNA and reducing the risk of disease.
- In summary, 8-OHdG is a critical biomarker for assessing oxidative damage to DNA, offering insights into the cellular impact of oxidative stress, its association with disease and aging, and the effectiveness of strategies aimed at reducing oxidative damage. Its measurement can provide valuable information for both clinical and research settings, underscoring the importance of managing oxidative stress for overall health and longevity.
- SST tube of blood
- Lavender tube of blood, EDTA
14 days
Price: $299.00